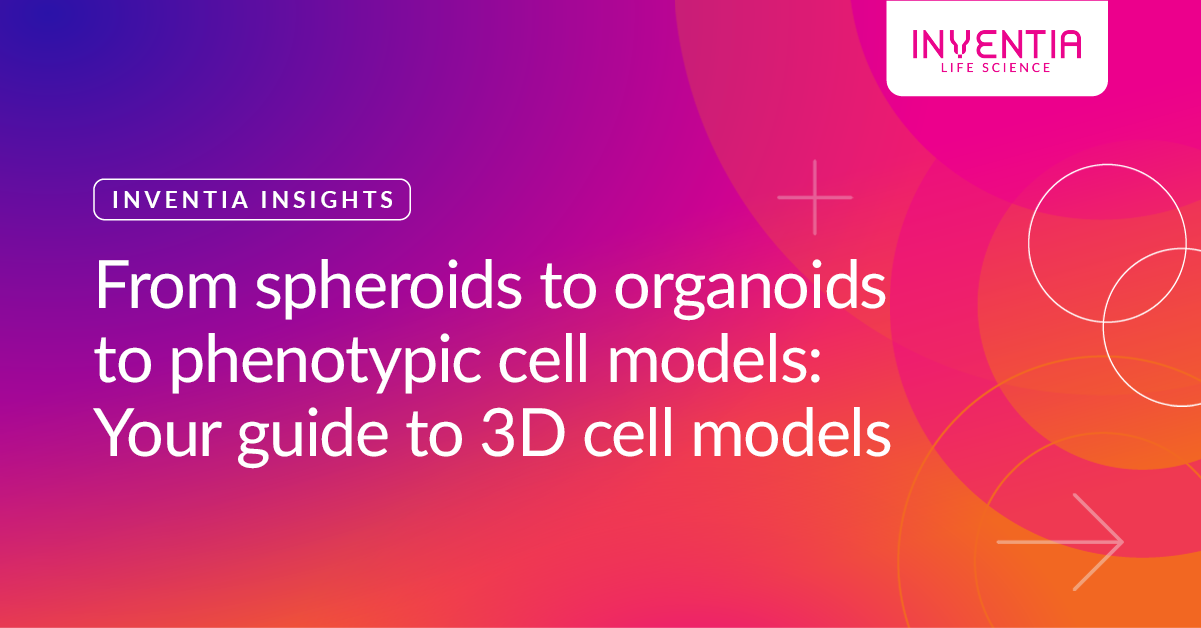
From spheroids to organoids to phenotypic cell models: Your guide to 3D cell models
In biological research, having the right tools isn’t just important—it’s transformative. When it comes to 3D cell models, terms like aggregates, spheroids, organoids, tumoroids, advanced cell models, and phenotypic models often seem like a jumble of jargon. Yet, these names represent essential building blocks for studying biology in ways that were previously out of reach.
Each type of model brings unique strengths and applications to the table, helping researchers bridge the gap between simple 2D cultures and the complexity of living tissues. By understanding the core characteristics of each model, you can lay the groundwork for breakthroughs in areas ranging from basic biology to personalized medicine.
The importance of 3D cell models
The history of 3D cell models traces its roots back to early tissue culture practices, predating the conventional 2D culture systems. In the late 19th and early 20th centuries, Robert Koch (1880) and Ross Harrison (1906) pioneered early tissue culture techniques by cultivating cells in their native three-dimensional contexts. They demonstrated the feasibility of maintaining living tissues outside the organism, laying the foundation for modern cell culture. These early experiments inherently embraced a 3D structure, reflecting the natural environment of cells. However, with the advent of 2D culture systems on rigid substrates like glass and plastic, researchers adopted simpler, more controllable methods for cell growth. As understanding of cellular complexity advanced, the limitations of 2D culture spurred a resurgence in 3D culture techniques. Today, modern 3D cell models, empowered by technologies like ultra-low attachment surface, hydrogels, and bioprinting, continue to build upon these historical milestones, replicating the complexity of tissues with unprecedented precision.
For two decades, 3D cell models have revolutionized biomedical research by providing a more physiologically relevant environment for various fields, from fundamental research to applied fields like personalized medicine and drug discovery, and have proven to be indispensable. Patient-derived models enable tailored treatment strategies, while advanced systems improve drug screening accuracy by better predicting human responses. Whether for understanding diseases, testing therapies, or accelerating innovation, these tools empower scientists to uncover deeper insights and deliver transformative solutions.
Cell aggregates: the cornerstone of 3D cell culture models
Cell aggregate is the generic term describing the clustering and adhesion of initially separate cells to form an aggregate. They offer a simple, yet powerful way to mimic the 3D organization of tissues. They are formed by the self-assembly of cells into clusters, driven by cell-cell adhesion and communication, closely replicating in vivo tissue microenvironments. Early use of cell aggregates dates back to the early 20th century, when Wilson, in 1907, demonstrated the possibility to reaggregate sponge single cells to form in vivo-like tissue structures. The 1950s saw the development of advanced cell models with the realization of 3D culture in matrices (here cellulose) that support tissue formation and the formation of 3D cultures on low attachment surfaces to form sphere-shaped aggregates called spheroids.
Spheroids: The foundation of 3D models
Spheroids are clusters of cells that naturally form into rounded structures. Their simplicity makes them easy to generate and highly versatile. Typically derived from primary cells of immortalized cells, they are grown in suspension or embedded in a supportive matrix. They are typically formed from one single cell type. However, multicellular spheroids loaded with different cell types can also be formed after culture medium optimization.
Simple yet powerful, spheroids are an accessible entry point for researchers venturing into 3D models. Since the 1950s, spheroids have excelled at replicating basic features of solid tumors, such as oxygen, nutrient, and waste gradients, assessing drug-induced toxicity, and investigating tissue development, degeneration/regeneration, physiology, and drug response. However, their simplicity also limits their ability to replicate the complexity of tissues or multi-cellular interactions.
Despite these limitations, spheroids are an essential starting point. They provide the foundational insight needed to build more advanced models as research questions grow more complex.
Organoids: The architects of complexity
Building on the foundation of spheroids, organoids offer a more advanced approach to 3D modeling. These self-organized systems capture key aspects of tissue structure and function, enabling researchers to study biological processes in greater detail.
Derived from stem cells, organoids differentiate into multiple cell types and organize into structures that resemble certain tissues, such as the intestine, brain, or liver. They provide a platform for studying development, disease progression, and tissue interactions. For example, brain organoids have been used to study neurodevelopmental disorders, while intestinal organoids enable research on nutrient absorption and microbiome dynamics.
In applied research, organoids are reshaping personalized medicine and drug discovery. Patient-derived organoids allow tailored therapeutic testing, offering a more predictive model for human responses. However, their complexity and resource-intensive requirements can limit scalability for certain applications.
Organoids represent a critical step forward in 3D modeling, enabling researchers to explore questions that simpler models like spheroids cannot answer.
Tumoroids: Capturing the complexity of cancer
Tumoroids, also known as tumor organoids, bring researchers closer to replicating the heterogeneity of real tumors. Unlike multicellular tumor spheroids (spheroids generated from established cancer cell lines under non-adherent conditions) developed by Sutherland in the 1970s, tumoroids derived from patient tumor cells. These models retain key genetic and structural features of the original tumor, making them a powerful tool for cancer research. They excel at mimicking patient-specific tumor characteristics, offering valuable insights into therapeutic efficacy by allowing researchers to test how treatments interact with models that closely resemble the patient’s tumor. Tumoroids also help unravel the mechanisms of tumor growth, invasion, and resistance, providing a deeper understanding of cancer biology. Furthermore, by preserving elements of the tumor microenvironment, these models enable the study of immune-tumor interactions, advancing the field of immunotherapy.
While challenges such as limited availability and variability persist, tumoroids bridge the gap between basic research and clinical applications. By capturing the complexity of cancer, they offer researchers a unique opportunity to study the disease in its full intricacy and develop more precise and effective therapies.
Pioneering the future of 3D cell culture
As biological questions become more complex, advanced cell models, including spheroids, organoids, tumoroids, and bioprinted tissues, offer a transformative approach. Despite their significant advantages over traditional 2D cell cultures, they have limitations that can impact their effectiveness and broader adoption, such as the lack of standardization and reproducibility, a limited long-term stability, an incomplete or uncontrolled extracellular matrix, and a low scalability. Recently, progress has been made to overcome those limitations, leading to the formation of more accurate cell models called phenotypic models.
What are phenotypic cell models?
Phenotypic models take advanced culture models to the next level by incorporating key aspects of the tissue microenvironment. They can include multiple cell types, spatial organization, and tunable matrices that reflect the stiffness, nutrient gradients, or signaling cues found in vivo. This additional complexity allows researchers to investigate not only what cells do, but how they behave in the context of their surroundings.
Phenotypic models, like those created with RASTRUM™, enable researchers to study cellular behavior in dynamic, matrix-embedded systems. These systems are tunable to reflect tissue stiffness, nutrient gradients, or signaling cues, making them ideal for investigating complex biological processes. They are designed to study cells based on their observable behaviors and interactions rather than molecular characterization alone. Using RASTRUM, these models can be created with unprecedented scalability and reproducibility. The platform enables the generation of matrix-embedded systems that provide physiologically relevant conditions, supporting diverse research needs.
Key features of phenotypic models produced by RASTRUM include:
- Tunable microenvironments: The matrix can be adjusted to reflect specific tissue stiffness or mechanical properties, making it suitable for a range of cell types and applications.
- Enhanced complexity: Models can include multiple cell types and spatially organized architectures, better mimicking the interactions and structures found in vivo.
- Scalability: RASTRUM enables the rapid and consistent production of models, addressing challenges of reproducibility and throughput in 3D cell culture.
Applications of phenotypic models
These models are transforming research in several areas:
- Disease mechanisms: Phenotypic models allow researchers to study how diseases progress in environments that closely mirror human tissues.
- Drug discovery and toxicology: By replicating physiologically relevant conditions, they provide better predictive power for drug screening, reducing the risk of failure in clinical trials.
- Immunotherapy research: Advanced cell models are valuable for exploring interactions between immune cells and tumor or tissue cells, accelerating the development of new therapies.
The future of 3D cell models
The evolution from spheroids to advanced phenotypic models reflects the growing need for more nuanced tools in research and discovery. By capturing the complexity of biological systems, 3D cell models empower researchers to uncover new insights, develop innovative therapies, and push the boundaries of science.
As platforms like RASTRUM continue to drive progress, the future of 3D cell culture promises even greater possibilities. These models are not just tools—they are the building blocks of breakthroughs that will define the next era of biology.