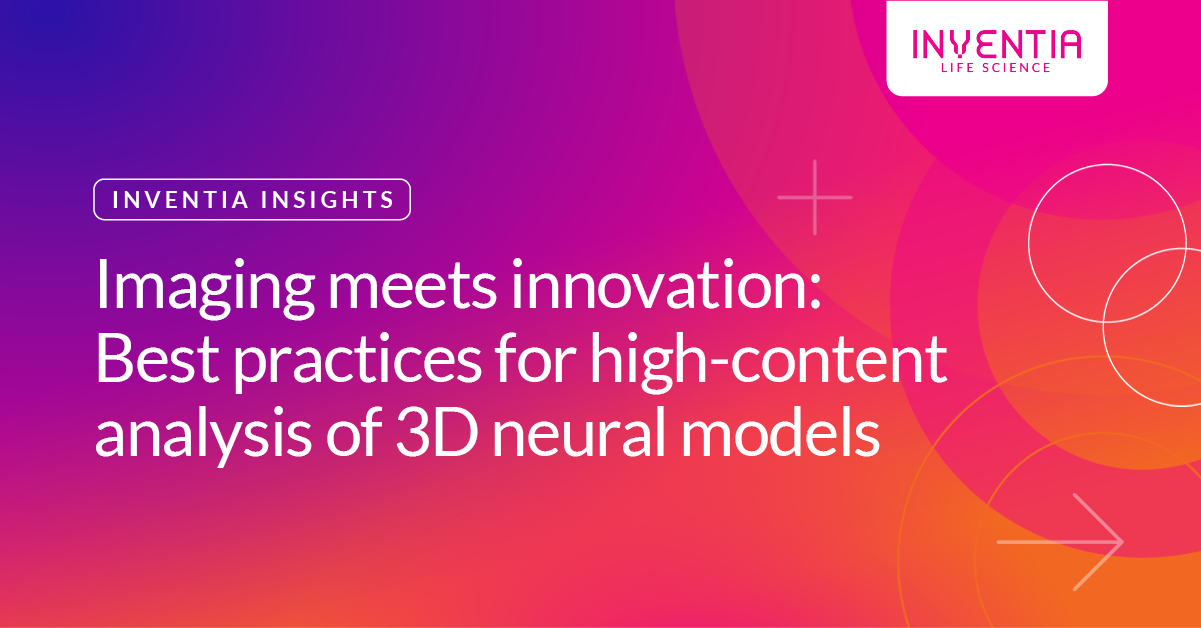
Imaging meets innovation: Best practices for high-content analysis of 3D neural models
Seeing beyond the surface
In science, breakthroughs often happen when we dare to look deeper. But for years, neuroscience research has been limited by the very tools designed to uncover the brain’s secrets.
Animal models—long considered the gold standard in neuroscience—offer valuable insight into brain development, function, and disease. But they also come with significant limitations: species-specific biology, ethical considerations, and the practical difficulty of dynamically studying neuronal networks in vivo. While these models can reflect the complexity of the brain, they’re often not ideal for higher-throughput experimentation or mechanistic dissection at the cellular level.
On the other end of the spectrum, in vitro 2D cultures—especially rodent-derived neuronal cultures, are more accessible and experimentally convenient. But this simplicity comes at a cost: neurons grow in flat monolayers, stripped of the three-dimensional context and architecture that shape their function in vivo. As a result, key features of neural networks—such as spatial organization, synaptic complexity, and matrix interactions—are poorly modeled, limiting the relevance of 2D systems for studying real brain behavior.
And in neuroscience, that missing third dimension isn’t just a limitation—it’s a barrier to understanding.
As advanced 3D neural models become more accessible, neuroscientists now have a powerful in vitro tool: one that balances biological complexity with experimental control. But this leap forward introduces new challenges—particularly in imaging. How do you visualize dense, multilayered networks without sacrificing resolution? How do you ensure reproducibility at scale?
This is where innovation meets execution.
By integrating Inventia Life Science’s RASTRUM™ 3D cell culture platform with Yokogawa’s CellVoyager™ high-content imaging systems, researchers are now able to generate, image, and analyze complex 3D neural models with confidence and clarity.
Reproducibility at the core: A 3D cell model built for imaging
Creating a 3D neural model is one thing—capturing consistent, high-quality data from it is another. RASTRUM solves both by offering biologically relevant, reproducible 3D models that are optically clear and optimized for fluorescent imaging.
“RASTRUM Matrices and cell model architectures are engineered for high content screening with low autofluorescence and tight z-distribution,” says Martin Engel, PhD, Principal Scientist at Inventia Life Science. “Every RASTRUM 3D cell model is printed with precision, ensuring consistent architecture and cell placement across wells and plates.”
These matrix properties address a real challenge for imaging scientists. “Some hydrogels or matrices fluoresce more than expected, and that creates a ton of noise,” explains Esther Kieserman, PhD, Senior Applications Specialist at Yokogawa. “This background can overpower the dim fluorescent signals in your sample that you're interested in.” Choosing a system like RASTRUM, where the matrix is optically clean and engineered for imaging, helps eliminate that variable right away. Combined with confocal imaging—which further reduces background by rejecting out-of-focus light—the result is a high signal-to-noise workflow ideal for fluorescence-based analysis.
Together, this low autofluorescence and structural consistency are what make RASTRUM models uniquely suited for high-content imaging. That level of reproducibility becomes especially important when transitioning to workflows where consistency directly impacts data quality. Dan Collins, Application Scientist at Yokogawa, emphasizes that, “the goal is to create an assay that is statistically relevant and can be used over time to generate quality results with minimal human intervention.” Getting there requires careful optimization—of staining protocols, label concentrations, incubation times, and more—to reduce variability and ensure that every assay delivers meaningful, reproducible results.
This approach was put into practice in a collaborative study between Inventia Life Science, Yokogawa, and the University of Sydney’s Halliday lab. Using RASTRUM, researchers generated 3D neural cultures with two different matrix compositions and analyzed MAP2 expression and branching behavior over 28 days using the CellVoyager CV8000 system.
The challenge of imaging 3D cell models
3D imaging introduces new dimensions—literally and technically. It requires rethinking acquisition strategies from the ground up.
“Phototoxicity and photobleaching are important factors to consider, especially in 3D imaging”, explains Esther. “In 2D, you expose the sample once per color. But in 3D, if you're capturing five z-slices with three channels, you’re exposing the sample 15 times. That increases the risk of photobleaching significantly.” If the assay involves a time series, this effect is, of course, exacerbated, and the effects of phototoxicity can even confound the treatment effect data.
Building an effective imaging setup means reworking standard protocols to suit 3D biology. Factors like pinhole spacing, Z-step size, and acquisition speed become even more critical when working with thicker, more complex samples.
In 3D imaging, it’s easy to get overwhelmed by complexity. That’s why Esther recommends taking a step back. “Pause, evaluate your experiment, and identify your top 2–3 priorities,” she says. “Then, adjust your parameters accordingly.” For instance, start by clearly defining your biological question, and determine whether full-structure imaging is necessary—or if a representative subset will do. As with all high-content assays, less can be more. Assessments in the validation stage should be focused on the minimum magnification, cell numbers and Z step numbers and intervals required to meet statistical thresholds and not just biological optimization (setting appropriate controls, dilutions series, etc.), to decrease imaging times and data storage requirements while increasing sample throughput.
Designing a workflow that “works”
Optimizing high-content imaging of 3D neural models means building workflows that are scalable, reproducible, and designed with longevity in mind.
“The goal is to create an assay that is statistically relevant and usable long-term with minimal manual intervention,” says Dan. To achieve that, researchers must carefully tune parameters like staining protocols, label concentrations, and incubation times to minimize variability and improve assay sensitivity.
Rigorous planning around experimental controls is just as important. “Where appropriate, positive and negative controls should be on every plate,” Dan explains. “Beyond experimental design, you want to normalize your fluorescent labeling, and optimize label selection, sampling size, magnification and the resolution needed. You need to think through the whole workflow.”
Dan encourages teams to invest early in assay development. “If you invest the time up front – doing test titrations, running pilot studies, using the Design of Experiments process – you’ll save a huge amount of troubleshooting later. And you’ll generate data that holds up under scrutiny.”
Even with automation in place, reproducibility depends on execution. Biology introduces variability—so it’s how you handle that biology, consistently and with intention, that makes the difference.
Choosing the right tools: RASTRUM + Yokogawa
Not all 3D models are created equal—and not all are imaging-ready. That’s why the combination of RASTRUM and Yokogawa offers a distinct advantage for high-content imaging.
“RASTRUM was designed with downstream workflow integration in mind,” says Martin. “From the optical properties of the matrix to the uniformity of the printed structures, everything is built to support clean, reliable imaging. When your models are consistent, it frees researchers to focus on generating meaningful biological insights.”
With the CellVoyager CV8000, that clarity becomes actionable. By using Yokogawa’s TargetSearch automated technique, researchers can intelligently target their imaging across large datasets. “Unlike a 2D field of confluent cells, 3D structures may not cover your entire plate or well…making it more difficult and time-consuming to locate the samples you're interested in,” explains Esther. “With TargetSearch you can scan the whole plate at low magnification, analyze for parameters like size, intensity, and location, and then go back and do high-resolution z-stacks just on the targets we care about. It’s a huge time and data storage saver.”
This targeted approach not only improves throughput and data quality—it reduces unnecessary light exposure, minimizes photobleaching, and ensures researchers are imaging the biology that matters most.
Avoiding common pitfalls in imaging 3D cell models
Even with the right tools, imaging 3D cell models can be tricky. Thick samples, complex architectures, and delicate fluorescent signals all make for a challenging environment. But, as the Yokogawa team emphasizes, many of the most common pitfalls are entirely avoidable—with the right setup and a little foresight.
One of the biggest issues researchers encounter is autofluorescence—especially from certain growth media, substrates, or 3D scaffolds. In low-signal applications, that background noise can easily overwhelm the biology.
“ECM autofluorescence is a big one,” says Tony Cooke, Account Manager, Yokogawa. “Some hydrogels or matrices fluoresce more than expected, and that creates a ton of noise.” Choosing a system like RASTRUM, where the matrix is optically clean and engineered for imaging, helps eliminate that variable right away.
Staining depth and dye penetration are another common hurdle. In 3D models, cells aren’t grown on a single plane—they’re 3-dimensionally adjacent in all directions, making it harder for antibodies or stains to reach their targets evenly.
“Staining needs to be verified during the formation of your spheroids or organoids,” Tony explains. “You want to confirm that your markers are getting in and that signal intensity is consistent throughout the structure—not just at the surface.”
Z-resolution and step size are critical parameters in 3D imaging. Oversampling increases data volume and slows acquisition without necessarily improving results, while under-sampling can lead to loss of important structural detail. The best approach depends on your specific imaging goals—whether you're aiming for accurate 3D reconstruction or extracting assay-relevant features through projections—and should be guided by both optical calculations and the biological question at hand.
Another hidden hazard? Saving the wrong data. Arvonn Tully, Senior Software Applications Specialist, Yokogawa, notes that some users default to saving only maximum intensity projections (MIPs) to reduce file size—but that can compromise downstream analysis.
“MIP images are helpful for morphology, but in larger 3D structures which have multiple layers, they can be misleading,” Arvonn explains. “You lose the depth information that matters most for accurate quantification. Slice-based analysis gives you much better insight into 3D morphology and the phenotypic distribution of cells.”
Even data storage itself becomes a consideration. “A single 3D assay can generate terabytes of data,” says Esther. “It’s critical to plan for that storage—not just for acquisition, but also for reanalysis later on.” For larger teams or high-throughput setups, this can place a real strain on data infrastructure and IT resources—making thoughtful planning around acquisition settings and file formats just as important as the imaging itself.
In other words, 3D imaging is more than a technical task—it’s a strategy. Avoiding common pitfalls requires not only smart tools, but smart habits: label validation, thinking critically about things like step size and image format, and by using automation wherever possible to eliminate human error.
“With 3D models, there are more moving parts—but there are also more opportunities to get it right,” says Martin. “If you plan ahead, use the right matrix, and take full advantage of what your imaging system can do, you’re setting yourself up for reproducible success.”
From imaging to insight
High-content imaging of 3D neural models unlocks more than just visualizations—it delivers insights into complex biological processes. The combination of reproducible 3D culture and intelligent imaging creates a foundation for deeper scientific understanding.
“In our study, we didn’t just see MAP2-positive neurons—we quantified branching complexity and architectural differences across matrix types,” says Martin.
This level of detailed analysis allows researchers to go beyond identification and launches the exploration of functional biological questions—from neurite outgrowth to cellular interactions within the matrix. With the right models and tools, subtle phenotypic shifts become measurable, and hypothesis-driven questions become quantifiable.
By combining a powerful 3D platform like RASTRUM with high-content imaging systems like the CellVoyager CV8000, researchers gain the sensitivity and scale required for applications in disease modeling, drug screening, and more.
A clearer path to discovery
In neuroscience, understanding starts with seeing clearly. The combination of reproducible, biologically relevant 3D cell models and high-performance imaging platforms offers researchers a new way forward—one that bridges biological relevance with data-driven discovery.
“There’s no one-size-fits-all answer in 3D imaging,” says Esther. “But if you stay thoughtful, stay flexible, and keep learning—you’ll get the data you need, and move your discoveries forward.”
With Inventia’s RASTRUM platform and Yokogawa’s CellVoyager CV8000, 3D imaging becomes more than a technical achievement—it becomes a foundation for discovery.
Ready to dive deeper? Download the full application note, Advanced neural models for high-content image analysis, to explore the full imaging workflow, quantitative results, and expert tips from the Inventia Life Science and Yokogawa collaboration.