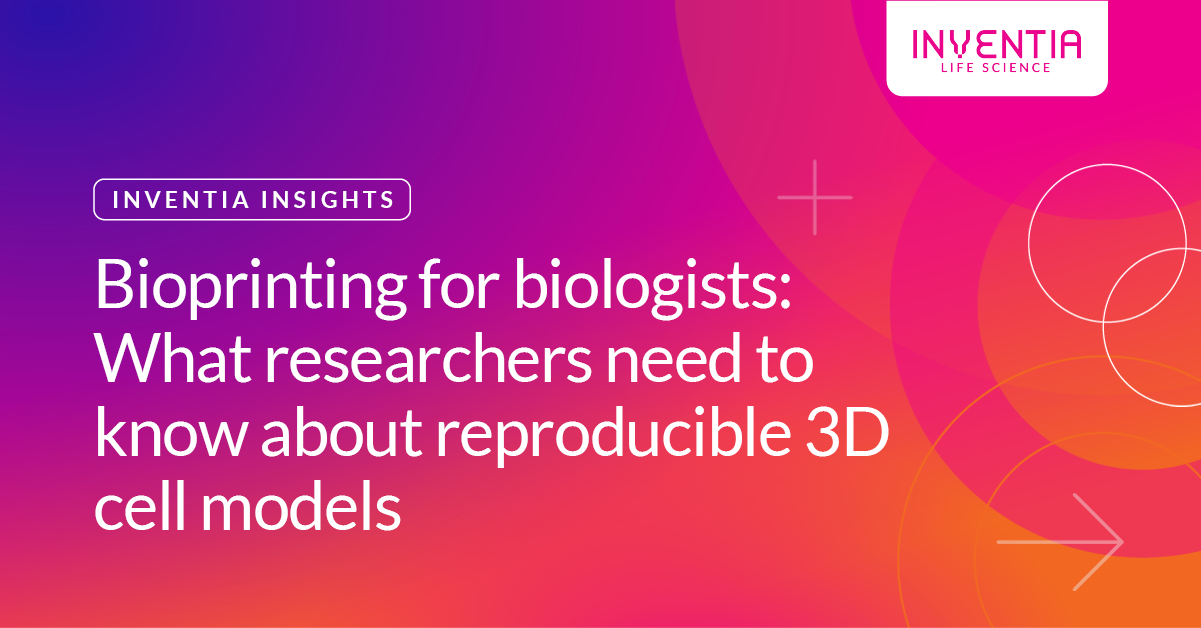
Bioprinting for biologists: What researchers need to know about reproducible 3D cell models
In drug discovery and disease modeling, 3D bioprinting isn’t about printing organs—it’s about building reproducible, high-throughput 3D cell models that researchers can trust. While headlines often focus on futuristic visions of bioprinted tissues and organs, the immediate need in biomedical research is far more practical: a scalable, consistent way to generate physiologically relevant 3D cell cultures.
Traditional methods like manual spheroid culture and animal-derived basement membrane embedding introduce variability, making it difficult to obtain reliable, reproducible data. This is where drop-on-demand bioprinting changes the game. By precisely depositing tiny droplets of bioink, drop-on-demand bioprinting enables scalable, automated 3D cell model production with the consistency required for high-throughput experiments and translational research.
In this article, we’ll explore why reproducibility matters, the differences between tissue engineering and 3D cell models, and how platforms like RASTRUM are transforming bioprinting into a practical tool for biological research.
What is bioprinting: How 3D bioprinting is transforming biomedical research
3D printing has become a mainstream technology, revolutionizing industries from manufacturing to healthcare. It’s easy to imagine a future where this same precision and automation can be leveraged in biology—printing cells, tissues, or even whole organs. But bioprinting is far more complex than just applying 3D printing to biology. Cells are living, dynamic, and require careful handling to maintain viability and function, introducing key challenges that must be addressed.
Key challenges in bioprinting
For bioprinting to deliver on its full potential as a standard tool in biological research, several critical factors must be addressed:
- Reproducibility: Biological systems are inherently variable. Generating consistent, high-throughput 3D cell models requires precise control over cell placement, matrix composition, and environmental factors.
- Cell viability: Unlike plastic or metal printing, bioprinting involves living cells that can be damaged by shear stress, temperature fluctuations, and mechanical forces during the printing process.
- Scalability: Many bioprinting methods are slow or labor-intensive, making it difficult to generate the large-scale, high-throughput cell models required for drug discovery and disease research.
- Biomaterial: Developing bioinks that replicate the mechanical, chemical, and biological properties of human tissues while supporting cell growth, adhesion, and differentiation, and maintaining printability.
To overcome these challenges, several bioprinting approaches have emerged, each with different advantages and limitations.
Major approaches to bioprinting
Bioprinting technologies fall into three major categories, each using different mechanisms to deposit cells and biomaterials:
- Extrusion-based bioprinting: The most common method, where bioinks (cells + hydrogels) are extruded through a nozzle. While versatile, this approach requires continuous bioink flow, which can affect cell distribution, viability, and structural integrity in 3D models.
- Laser-assisted bioprinting: Uses focused light to precisely position cells and biomaterials. This method provides high resolution, but is slow, expensive, and less scalable for routine applications.
- Droplet-based bioprinting: Similar to inkjet printing, this method deposits tiny droplets of bioink in a controlled, precise manner, making it gentler on cells and highly reproducible for large-scale applications.
Tissue engineering vs. 3D cell models for research: Two different goals
At first glance, the idea of bioprinting often conjures images of printing functional organs and implantable tissues—a future where bioprinted hearts and kidneys could solve the transplant crisis. But for many researchers, the true impact of bioprinting is already happening in a different space: drug discovery, disease modeling, and high-throughput screening.
Instead of engineering tissues for clinical implantation, biologists need reliable, reproducible 3D cell models that can mimic aspects of human biology in vitro. These models serve as testbeds for drug development, providing more physiologically relevant data than traditional 2D cultures. While both tissue engineering and 3D cell modeling use bioprinting, the technological requirements and end goals are vastly different.
In tissue engineering, the focus is on constructing intricate, multi-layered structures that can survive and function like native tissue, often using extrusion-based bioprinting to deposit thick layers of bioink. These constructs require complex support systems to mimic blood flow, oxygen delivery, and structural mechanics—challenges that make clinical applications decades away from reality.
In contrast, researchers working on drug screening, cancer biology, and disease modeling have a more immediate and practical goal: to create high-throughput, biologically relevant 3D cell cultures that provide more accurate insights than traditional 2D cell culture. They need these models to be consistent, scalable, and biologically relevant for screening drugs and understanding disease mechanisms.
That’s why scientists are turning to drop-on-demand bioprinting—a technology that offers precision, scalability, and reproducibility in 3D cell model generation.
For biologists in drug discovery, bioprinting isn’t about transplantable organs—it’s about revolutionizing how we study diseases and test new therapies—right now.
The advantages of drop-on-demand bioprinting for 3D cell culture
Bioprinting isn’t just about placing cells into a 3D structure—it’s about controlling how they’re placed to ensure reproducibility, scalability, and biological relevance. Drop-on-demand bioprinting is a technology designed for exactly that.
At its core, drop-on-demand bioprinting works similarly to inkjet printing, but instead of depositing ink, it dispenses tiny droplets of bioink containing cells and matrix components. Each droplet is carefully placed, allowing for high precision, minimal cell stress, and reproducible 3D cell model formation. Unlike traditional methods that rely on bulk extrusion or manual pipetting, drop-on-demand technology enables rapid, automated, and controlled bioprinting, making it particularly well-suited for biologists focused on disease modeling and drug discovery.
How drop-on-demand bioprinting works
Drop-on-demand bioprinting operates by ejecting nanoliter-scale droplets of bioink from a printhead to build a 3D cell model. Each droplet is placed with high accuracy, ensuring consistent cell model dimension, cell distribution, and matrix composition.
One of the defining features of drop-on-demand bioprinting is its ability to print multiple components simultaneously. Unlike manual cell culture methods, where embedding cells can be labor-intensive and inconsistent, drop-on-demand bioprinting ensures that cells are precisely encapsulated in a controlled microenvironment—with the right matrix stiffness, biochemical cues, and spatial organization.
This level of control matters. Cell behavior is highly dependent on its microenvironment, and drop-on-demand bioprinting makes it possible to fine-tune these conditions while maintaining high cell viability and reproducibility across experiments.
Learn more about drop-on-demand technology in this application note: Drop-on-demand printing technology of the RASTRUM 3D cell culture platform.
Why drop-on-demand bioprinting is transforming 3D cell culture
The advantages of drop-on-demand bioprinting go beyond simply placing cells in 3D space—it is a fundamentally different approach to building biologically relevant models.
Gentle on cells, high viability
- Drop-on-demand bioprinting minimizes shear stress, gently deposits cells without damaging them to preserve cell health.
- Leads to more representative 3D cultures, improving experimental reliability and biological relevance.
Explore how RASTRUM Allegro can help you maximize the potential of your precious cells.
Unparalleled reproducibility
- Ensures precise droplet placement, so each 3D model is built identically well-to-well, plate-to-plate, and lab-to-lab.
- Reduces experimental variability, making results more consistent and repeatable.
- Enhances data reliability in drug screening and disease modeling, ensuring meaningful insights.
Rapid, high-throughput 3D cell model generation
- Optimized for speed and scalability, enabling the creation of hundreds of 3D cell models in minutes.
- Streamlines large-scale experiments, making high-content screening and reproducibility studies more efficient.
- Eliminates the time constraints of manual culture methods, accelerating research timelines.
Explore how RASTRUM Allegro can help you create scalable and reproducible 3D cell models in this application note: Scalability and reproducibility for high-throughput biological modeling in 3D cell culture.
Multi-component control for complex models
- Mimics in vivo biology by enabling precise direct and indirect co-culture cell models.
- Allows multiple bioinks to be printed simultaneously, creating complex microenvironments.
- Supports tunable matrix properties, ensuring better control over cell interactions and tissue structure.
Explore RASTRUM cell model architectures to see how you can easily integrate established architectures into your workflows and a publication from MSD/Merck on creating an Alzheimer’s Disease model with the triple matrix architecture: Investigating Connectivity Deficits in Alzheimer’s Disease Using a Novel 3D Bioprinted Model Designed to Quantify Neurite Outgrowth.
Why drop-on-demand technology matters for biologists
For biologists working in drug discovery and disease modeling, drop-on-demand bioprinting isn’t just an incremental improvement—it’s a paradigm shift. It eliminates the inconsistencies of manual methods, overcomes the limitations of extrusion-based techniques, and provides a reliable way to generate high-quality 3D cell cultures at scale.
By combining precision, reproducibility, and high cell viability, drop-on-demand bioprinting is making 3D cell culture a practical, scalable tool for research—one that is already transforming the way scientists study diseases and develop new therapies.
RASTRUM: A platform for reproducible and scalable 3D cell models
For researchers working in drug discovery and disease modeling, the promise of bioprinting isn’t just about creating 3D structures—it’s about generating reliable, reproducible models that can drive real scientific insights. That’s exactly what RASTRUM delivers.
What Sets RASTRUM Apart?
RASTRUM is a high-throughput drop-on-demand bioprinter purpose-built for 3D cell model generation. Unlike traditional bioprinters, which often struggle with variability, scalability, and speed, RASTRUM is designed to seamlessly integrate into biological research workflows, providing precision, reproducibility, and efficiency at scale.
RASTRUM:
-
Uses synthetic bioinks that gel instantly, allowing precise control over cell environments and matrix conditions.
-
Enables high-throughput screening, making it an ideal tool for both pharmaceutical and academic research.
-
Delivers unprecedented reproducibility, ensuring consistent results experiment to experiment, lab to lab.
With RASTRUM, researchers can eliminate the inconsistencies of manual culture methods and achieve biologically relevant 3D models—faster and more reliably than ever before.
Case study: High-throughput drug screening with RASTRUM
A recent study presented at SLAS 2025 showcased the capabilities of the RASTRUM platform in developing reproducible, high-throughput 3D models for pancreatic ductal adenocarcinoma (PDAC): Development of a reproducible, high-throughput, and screenable 3D PDAC model using the RASTRUM™ platform. These models were utilized to evaluate both established chemotherapeutic agents and novel therapeutic candidates.
Key findings:
-
High-throughput capability: The RASTRUM platform enabled the generation of 3D PDAC cell models across 30+ plates in a single day.
- Reproducibility and consistency: The models exhibited characteristic 3D morphology and consistent viability with minimal variation across multiple plates, underscoring the platform's reproducibility.
- Drug response evaluation: The PDAC models responded to a subset of test compounds, demonstrating the platform's effectiveness in assessing drug toxicity and efficacy.
These findings highlight the importance of utilizing reproducible, scalable 3D cell models—such as those enabled by RASTRUM™—in modern drug discovery efforts.
Conclusion: Bioprinting that works for biologists
3D bioprinting is no longer just about the future—it’s about what’s possible today. The ability to generate scalable, reproducible 3D cell models is transforming how researchers study diseases, test drugs, and develop therapies. Start building scalable, reproducible 3D cell models with RASTRUM. Learn how the RASTRUM platform can accelerate your research in drug discovery and disease modeling.