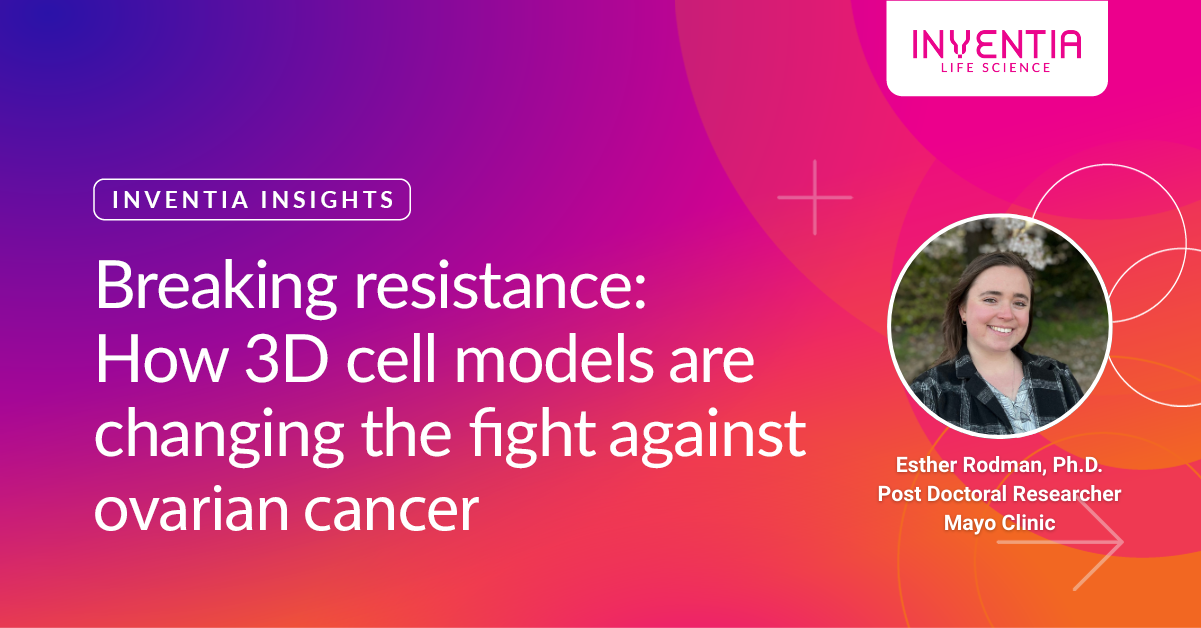
Breaking resistance: How 3D cell models are changing the fight against ovarian cancer
A scientist on a mission
For Esther Rodman, PhD, science has always been about impact. During her PhD studies at the Mayo Clinic, working in the laboratory of John R. Hawse, PhD, she focused on one of the biggest challenges in oncology: drug resistance in ovarian cancer.
“Patients in the clinic right now are developing resistance to whatever drugs we give them,” Esther explains. “Until we can prevent or cure ovarian cancer, there’s always going to be a need for new treatments. Unfortunately, cancer cells are resilient, they figure out how to become resistant to anything we throw at them.”
Ovarian cancer remains one of the deadliest cancers, largely because of its ability to develop resistance to therapy.
“Over 75% of ovarian cancer patients experience recurrence following their frontline therapy,” Esther explains. “And for a large number of those patients, their recurrent disease is resistant to further treatment with those therapies.”
Once resistance sets in, options become limited, and patients enter a distressing cycle: response, recurrence, response, recurrence—each time the cancer can become even more resistant and to an increasing number of therapies. Even the introduction of new drug classes, such as PARP inhibitors, hasn’t been enough to break this pattern.
“We don’t really have options for them,” Esther says. “Even though we’ve added PARP and VEGF inhibitors to the list of FDA approved therapies, the majority of ovarian cancer patients experience this cycle.”
Faced with this challenge, Esther’s research set out to identify a novel compound that could be effective against resistant ovarian cancer.
The shift to 3D: A turning point
Traditional drug testing relies on 2D cell cultures—cells grown on flat plastic surfaces. But as Esther’s research progressed, it became clear that something was missing.
“We’ve seen so many drugs fail when we move from in vitro cell lines in the lab to mice and then further into patients. Many scientists are constantly striving to make our drug testing more relevant. For example, people are 3D. We’re not growing on plastic. There are many non-cancerous cells that are components of a tumor” Esther says.
“There was one paper that asked a very simple question: that is, how do ovarian cancer cells change when you grow them in 2D on plastic, or in suspension in ultra-low attachment conditions, or when they are embedded in Matrigel which is used to mimic the extracellular matrix,” she says. “They conducted a meta-analysis of all the papers out there that had RNA sequencing or microarray data comparing 2D vs 3D growth and there were a number of signaling pathways differences that came out of that study.”
But not all 3D models are created equal. Many traditional methods, like organoids and spheroids, lack reproducibility and scalability—both essential for drug screening. That’s where RASTRUM came in.
With RASTRUM, Esther’s team could consistently recreate tumor models and study drug responses at scale—allowing them to uncover patterns of resistance that would have otherwise gone undetected.
Discovering a new approach
With 3D models in place, Esther and her team screened potential drugs and identified a JAK/STAT inhibitor called lestaurtinib that potently killed sensitive and resistant forms of ovarian cancer.
“We identified it in a drug screen, and that’s what most of my thesis work focused on,” she explains.
“We discovered that resistant forms of ovarian cancer have vast differences in the genes that they’re expressing and the pathways that they rely on for survival,” she says. “One major difference we found was that ovarian cancer cells upregulate JAK/STAT signaling as they become resistant to chemotherapy and PARP inhibitors.”
“Given our interest in this inflammatory pathway, we were really interested in studying this drug in a 3D context,” Esther says.
But there was a catch. A clinical trial had already been conducted using another JAK/STAT inhibitor—ruxolitinib—and it failed.
“In that clinical trial, they did not see any clinically significant response,” Esther notes. “However, we have tested ruxolitinib in our lab, and it does not kill ovarian cancer cells at clinically relevant concentrations. Our studies predicted that this trial was not going to work to start with.”
The difference? Ruxolitinib was too targeted. Lestaurtinib, in contrast, was a broader inhibitor.
She found that RASTRUM printing of ovarian cancer cells in a 3D environment with relevant ovarian tumor extracellular matrix (ECM) components and stiffnesses dramatically induced JAK/STAT pathway activity, effects that were not observed with traditional 2D and 3D culture systems. This increased activity was associated with robust phosphorylation of two specific amino acid residues on STAT proteins.
“Interestingly, lestaurtinib was able to inhibit both of those residues,” Esther explains, “instead of just one as was the case for ruxolitinib”.
“It kind of gets us into this exciting—or unexpected—finding that a super-targeted inhibitor may not be the best. More of a broad, coming-at-things-from-multiple-angles approach might be better, at least in this model system.”
This unexpected discovery underscored a fundamental truth in cancer research: context matters.
What’s next? The future of cancer research in 3D
With the ability to generate highly reproducible 3D tumor models, Esther’s team is now focused on adding even greater complexity to better reflect real patient tumors.
“We’re working on how to add more layers of complexity—adding primary cells, particularly cancer-associated fibroblasts (CAFs),” she says. “Because we know they modulate the ECM and chemotherapy responsiveness. We found that when we grow ovarian cancer cells with CAFs in 2D, the CAFs do not promote chemotherapy resistance, but in 3D, they do.”
Beyond CAFs, the team is exploring how factors like collagen composition, immune cell interactions, and extracellular matrix remodeling shape tumor behavior. By mimicking the tumor microenvironment more closely, they aim to capture the complexity of real patient tumors and their responses to therapy.
In the future, this approach could help personalize therapy, allowing researchers to test multiple drugs on a patient’s own tumor cells before making a treatment decision.
“What’s really exciting is that you could have 96 different replicates of the patient’s tumor that’s recapitulated, that’s uniform, and actually do a drug screen on a patient tumor,” Esther says.
By enabling high-throughput screening with controlled 3D environments, RASTRUM allowed the team to detect patterns of drug resistance, and drug vulnerabilities, that would have otherwise gone unnoticed. This approach could one day help personalize treatment strategies by testing a patient’s own tumor cells to inform the most effective therapy.
Advice for scientists considering 3D cell models
For researchers hesitant to make the switch from 2D to 3D, Esther has some advice.
“I understand the hesitation,” she says. “It involves resetting a protocol. You might be worried that what you’re studying won’t be relevant anymore.”
But for her, the decision was clear.
“If I moved my models to 3D and I found none of my drugs work anymore, why would I continue working on those drugs?” she asks. “What’s the point if it’s not going to help a patient?”
She also emphasizes the importance of planning ahead.
“You’ve got to make sure you have a system set up to analyze models in 3D,” she says. “Because you can gain so much information—but if you don’t have a setup ready to go, you’re just going to kind of look at it and go, ‘Oh, this is cool. Now what?’”
Why it matters
At its core, Esther’s research isn’t just about science—it’s about people.
“If we can build better models, that’s really great for us,” she says. “Because we want to know: Is our drug going to work in a patient?”
With 3D cell models and platforms like RASTRUM, the answer to that question is becoming clearer than ever before.
And for the patients who need new treatments the most, that could make all the difference.